Is it possible to predict the ice temperature and its thermodynamic properties? In principle it would not seem difficult. The heat propagation equation has long been known. It therefore seems that it is enough to know the incoming solar radiation, have a simple model of heat propagation, a digital model of elevation of the glacier and the terrain, and that's it. There are some difficulties though. Assuming the physics works as described, we can understand fairly quickly that the heat capacity of the ice is enormous and if the mass of the glacier is large, this requires that the heat balance model must be run for hundreds of years to obtain reliable results. This is impractical because we would need to know the (forcing) meteorological conditions starting from a distant past (everyone knows that the future is unknown, fewer are those who reflect on the fact that not even the past and the present are perfectly known). In fact, as any mathematician knows, glacier modeling requires you to set the temperature and heat flow conditions around the glacier at any time (the boundary conditions). We do not have them but we can invent plausible ones, making use of a little art and a lot of artisan’s experience. This, to be honest, introduces some uncertainty into what is being built, but it would be enough to be clear about this and people could perhaps understand.
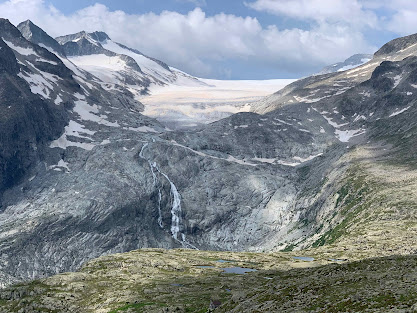
Obviously, as you can imagine, the thermodynamics of glaciers is not as trivial as we assumed at the beginning. It is a fact that knowledge of ice physics hasn't evolved much in the last twenty years or so. How does snow compact into ice? And how does ice behave under great pressure? How does the heat capacity of ice vary during these metamorphisms? And how the thermal conductivity? (The first factor tells how much energy it takes to make the temperature of a kilogram of ice vary by one degree, the second how fast, the energy changes redistribute through the ice pack). And, furthermore, what is the thermal variability of ice in space? The action that determines these quantities is called the characterization of the thermal properties of ice. This can be done by monitoring glaciers and making some assumptions, but it cannot be obtained without careful application.
In reality we should not only solve the thermal balance but the whole energy balance, which includes the possible phase transitions that generate the transformation of ice into water and water vapor and, of course, vice versa. If we make these necessary refinements, the equations become more difficult to be solved (well, some colleagues have said that for certain reasons the energy budget models are often wrong - not ours actually - due to the numerical consequences introduced by these aspects). When liquid water is added to ice, percolation and runoff are obviously obtained, which change the nature of the phenomena and of the descriptive equations, to which the advection-dispersion terms must be added.
Perhaps I also forgot to mention that snow is different from ice and the properties of the ground beneath the glacier are important in determining the general state of a glacier itself.
Going to the problem of the failure of the Marmolada glaciers, the failure itself is a different business because it has to deal with the formation of cracking and geo-mechanics of solids. They certainly depend on the thermodynamics but this connection itself is not easy to obtain since both the geometry of cracks and the mechanics are two further differentiated issues.
So do we have to conclude that the hope of modeling the thermodynamics of a glacier is a lost battle? Not at all! We must conclude that with the investigators patient, a lot of evidence can be gathered and some results can be obtained to get a decently approximate thermal state of the glacier. We can realize that above all it is an accumulation of information that is necessary and that this derives from the possibility of continuing research over time. Only patient application (and some intuition) eliminates the gray area in our knowledge of these problems and improves the models. Maybe we are year zero, but next year we could be year one, and so on.
For who wants to go a little deeper on the topic they can find a little of literature below.
References
Aschwanden, Andy, Ed Bueler, Constantine Khroulev, and Heinz Blatter. 2012. “An Enthalpy Formulation for Glaciers and Ice Sheets.”
Journal of Glaciology 58 (209): 441–57.
https://doi.org/10.3189/2012JoG11J088.
Beniston, Martin, Daniel Farinotti, Markus Stoffel, Liss M. Andreassen, Erika Coppola, Nicolas Eckert, Adriano Fantini, et al. 2018. “The European Mountain Cryosphere: A Review of Its Current State, Trends, and Future Challenges.”
The Cryosphere 12 (2): 759–94.
https://doi.org/10.5194/tc-12-759-2018.
Carletti, Francesca, Adrien Michel, Francesca Casale, Alice Burri, Daniele Bocchiola, Mathias Bavay, and Michael Lehning. 2022. “A Comparison of Hydrological Models with Different Level of Complexity in Alpine Regions in the Context of Climate Change.”
Hydrology and Earth System Sciences 26 (13): 3447–75.
https://doi.org/10.5194/hess-26-3447-2022.
Corripio, Javier González. 2002. “Modelling the Energy Balance of High Altitude Glacierised Basins in the Central Andes.” University of Edinburgh.
Endrizzi, S., S. Gruber, M. Dall’Amico, and R. Rigon. 2014. “GEOtop 2.0: Simulating the Combined Energy and Water Balance at and below the Land Surface Accounting for Soil Freezing, Snow Cover and Terrain Effects.”
Geoscientific Model Development 7 (6): 2831–57.
https://doi.org/10.5194/gmd-7-2831-2014.
Gouttevin, I., M. Lehning, T. Jonas, D. Gustafsson, and M. Mölder. 2015. “A Two-Layer Canopy Model with Thermal Inertia for an Improved Snowpack Energy Balance below Needleleaf Forest (model SNOWPACK, Version 3.2.1, Revision 741).”
Geoscientific Model Development 8 (8): 2379–98.
https://doi.org/10.5194/gmd-8-2379-2015.
Greve, R. and Blatter, H.: Comparison of thermodynamics solvers in the polythermal ice sheet model SICOPOLIS, Polar Science, 10, 11–23, 2016
Hewitt, I. and Schoof, C.: A model for polythermal ice incorporating gravity-driven moisture transport, Journal of fluid mechanics, 797, 2016
Hanzer, Florian, Kristian Förster, Johanna Nemec, and Ulrich Strasser. 2017. “Projected Cryospheric and Hydrological Impacts of 21st Century Climate Change in the Ötztal Alps (Austria) Simulated Using a Physically Based Approach.”
Hydrology and Earth System Sciences Discussions, August, 1–34.
https://doi.org/10.5194/hess-2017-309.
Hock, Regine. 2005. “Glacier Melt: A Review of Processes and Their Modelling.” Progress in Physical Geography 29 (3): 362–91.
Lehning, Michael, Perry Bartelt, Bob Brown, Charles Fierz, and Pramod Satyawali. 2002. “A Physical SNOWPACK Model for the Swiss Avalanche Warning.”
Cold Regions Science and Technology 35 (3): 147–67.
https://doi.org/10.1016/s0165-232x(02)00073-3.
Lehning, Michael, Ingo Völksch, David Gustafsson, Tuan Anh Nguyen, Manfred Stähli, and Massimiliano Zappa. 2006. “ALPINE3D: A Detailed Model of Mountain Surface Processes and Its Application to Snow Hydrology.”
Hydrological Processes, IAHS Publica, 20 (10): 2111–28.
https://doi.org/10.1002/hyp.6204.
Machguth, Horst, Frank Paul, Martin Hoelzle, and Wilfried Haeberli. 2006. “Distributed Glacier Mass-Balance Modelling as an Important Component of Modern Multi-Level Glacier Monitoring.”
Annals of Glaciology 43: 335–43.
https://doi.org/10.3189/172756406781812285.
Pellicciotti, Francesca, Marco Carenzo, Jakob Helbing, Stefan Rimkus, and Paolo Burlando. 2009. “On the Role of Subsurface Heat Conduction in Glacier Energy-Balance Modelling.”
Annals of Glaciology 50 (50): 16–24.
https://doi.org/10.3189/172756409787769555.
Perona, P., and P. Burlando. 2008. “Mechanistic Interpretation of Alpine Glacierized Environments: Part 1. Model Formulation and Related Dynamical Properties.” Advances in Water Resources 31 (June): 937–47.
Tiel, Marit, Kerstin Stahl, Daphné Freudiger, and Jan Seibert. 2020. “Glaciohydrological Model Calibration and Evaluation.”
WIREs Water 66 (September): 249.
https://doi.org/10.1002/wat2.1483.
Tubini, Niccolò, Stephan Gruber, and Riccardo Rigon. 2021. “A Method for Solving Heat Transfer with Phase Change in Ice or Soil That Allows for Large Time Steps While Guaranteeing Energy Conservation.”
The Cryosphere 15 (6): 2541–68.
https://doi.org/10.5194/tc-15-2541-2021.
Vionnet, V., E. Brun, S. Morin, A. Boone, S. Faroux, P. Le Moigne, E. Martin, and J-M Willemet. 2012. “The Detailed Snowpack Scheme Crocus and Its Implementation in SURFEX v7.2.”
Geoscientific Model Development 5 (3): 773–91.
https://doi.org/10.5194/gmd-5-773-2012.
Weiler, Markus, Jan Seibert, and Kerstin Stahl. 2018. “Magic Components-Why Quantifying Rain, Snowmelt, and Icemelt in River Discharge Is Not Easy.”
Hydrological Processes 32 (1): 160–66.
https://doi.org/10.1002/hyp.11361.
Wever, N., C. Fierz, C. Mitterer, H. Hirashima, and M. Lehning. 2014. “Solving Richards Equation for Snow Improves Snowpack Meltwater Runoff Estimations in Detailed Multi-Layer Snowpack Model.”
The Cryosphere 8 (1): 257–74.
https://doi.org/10.5194/tc-8-257-2014.
Zemp, Michael, Wilfried Haeberli, Martin Hoelzle, and Frank Paul. 2006. “Alpine Glaciers to Disappear within Decades?”
Geophysical Research Letters 33 (13): 303.
https://doi.org/10.1029/2006GL026319.
No comments:
Post a Comment